For thousands of years, olive oil production has been central to many different agricultural practices, spanning diverse regions in primarily temperate climates around the world. The cultivation of olive trees and the creation of olive oil have become more than just agricultural activities; they are woven into the cultural identities and economies of the places where olives thrive. Nowhere is this more evident than in the Mediterranean, where the olive tree has stood as a symbol of peace for centuries.
Today, the changing climate affects us all, even threatening the crops that have sustained us for centuries. Atmospheric carbon dioxide levels are projected to nearly double by 2050, resulting in an average temperature increase of 4.4 degrees Celsius by the end of the 21st century. Over 53 percent of the olive-growing regions along Turkey’s Aegean Coast—which accounts for 80 percent of the country’s total olive production—could become unsuitable for cultivating olive trees by 2050. This is a significant concern, as Turkey ranked among the top three countries in the world for olive and olive oil production between 2023 and 2024.
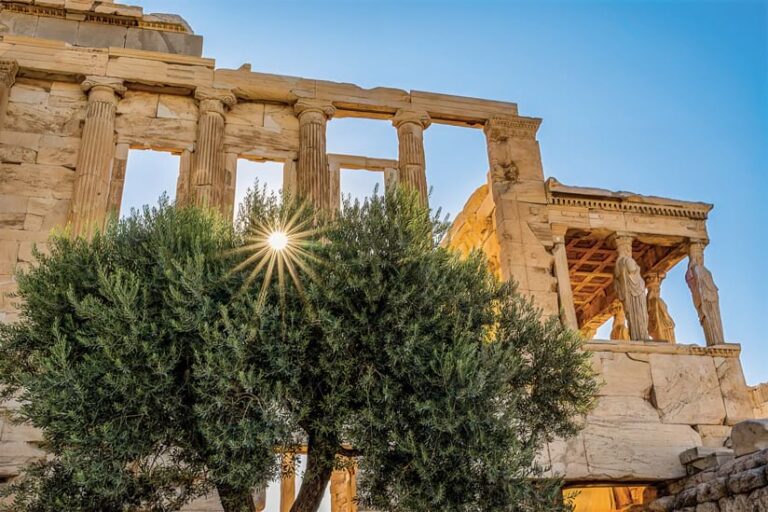
With the help of GIS technology, including ArcGIS Living Atlas of the World layers, we can pinpoint these vulnerable regions and assess the potential impacts of a changing climate on olive trees. GIS empowers us to take proactive measures to understand the cultivation of olives and other crops in those vulnerable regions and ensure the sustainability of this vital industry.
To address these challenges, we need to determine where to allocate resources and efforts by understanding the potential impact of climate change on terrestrial ecosystems by 2050 and how it will affect olive trees in those ecosystems. In order to gain a greater understanding of this impact, we decided to analyze the olive trees on Turkey’s Aegean Coast using ArcGIS Living Atlas.
New Threats to Old Vintages
Thousands of years after the first olive trees appeared on the Greek Aegean Coast, the family of Deniz Karagulle’s grandparents moved along the coast from Greece to Turkey in 1923 and established an olive farm in Izmir. Since then, the family has produced its own olive oil and olives from that farm—until 2023.
In October 2023, during the harvest season, Karagulle visited her grandparents and joined them on the farm to pick olives. As they worked, Karagulle asked her grandfather how the farm was doing compared to previous years.
Things weren’t going well. He explained that from 10 kg of olives, he used to extract 5 kg of olive oil, but now only gets 1 kg. He said the change is due to a lack of rain, as well as weather that’s much hotter than previous years.
According to Nicolas Netien, an environmental engineer and organic farming expert who cofounded Atsas Organics, the heat isn’t the issue. “The olives can withstand it,” he said. “The real challenge now is the shifting [weather] patterns. Conditions are becoming increasingly unpredictable, which means we need systems that are more adaptable and resilient.”
Each year, it is becoming harder to predict what will happen. Winter rains are not arriving, spring is experiencing unexpected snowfall during the flowering period, and intense heat comes a month early and lasts twice as long as it used to. All of this has placed significant stress on the olives, especially in 2024.
As the Food and Agriculture Organization of the United Nations noted, “Changes in temperature, precipitation, and extreme weather events due to climate change are already affecting crop yields and quality in many regions, threatening global food security.” This highlights how shifts in climate variables like temperature and precipitation can impact agricultural productivity and quality.
When climate shifts affect the surrounding ecology, it signals the need for adaptation. Otherwise, people like Karagulle’s grandparents begin to lose both the volume and quality of their crops. Olive farmers in Greece, Spain, Italy, California, and many other areas face similar challenges.
To understand the percentage of olive trees that may be impacted by changing climate and ecosystems, we performed spatial analysis using data from ArcGIS Living Atlas. You can implement the same method for your own region with any type of crops.
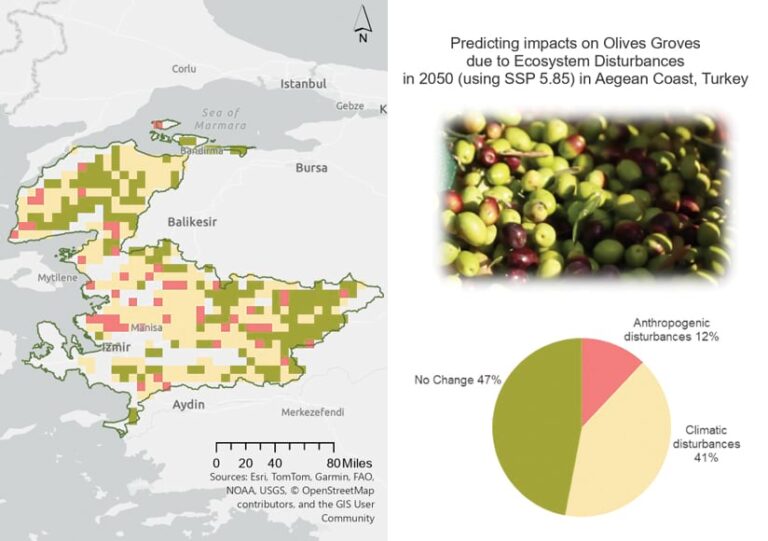
The Analysis Workflow
In our analysis, we used the 1 km resolution Projected Change Types for World Terrestrial Ecosystems (WTEs) in 2050, under the SSP5-8.5 scenario available in ArcGIS Living Atlas. This layer is derived from the Projected World Terrestrial Ecosystems for 2050 (SSP5-8.5) and World Terrestrial Ecosystems 2015 datasets. These datasets are available in ArcGIS Living Atlas, alongside a 10 km resolution olive tree dataset from International Food Policy Research Institute’s Spatial Production Allocation Model (SPAM).
By analyzing the 2015 and projected 2050 WTEs (see page 51), we observed potential global climate changes in ecosystem types. This analysis forms the basis of our study, using the Projected Change Types for WTEs in 2050 (SSP5-8.5) layer. This layer shows the types and combinations of modeled changes to WTEs from 2015 to 2050 under the SSP5-8.5 emissions scenario. We used this data to identify the drivers of ecosystem change in our model and to calculate the impact on olive trees within those drivers.
Ecosystem changes in our model are driven by climate-related disturbances and anthropogenic (human-caused) disturbances. Each WTE is defined as an area with a unique combination of the three inputs: climate setting, landform, and land cover. If any of the three input variable classes change, the ecosystem type itself changes.
To develop solutions for sustainable olive production and better land management planning, it’s essential to first identify where these changes are occurring. We created a set of change layers that indicate whether a change occurs and, if so, what type of change occurs. We used these layers to determine the percentage of olive trees located within each of the different types of ecosystem disturbance areas in 2050.
The analysis workflow involved reclassifying the change layers to identify areas of no change, change by anthropogenic disturbances, and change by climate disturbances. We then calculated the percentages of olive trees within these disturbance zones.
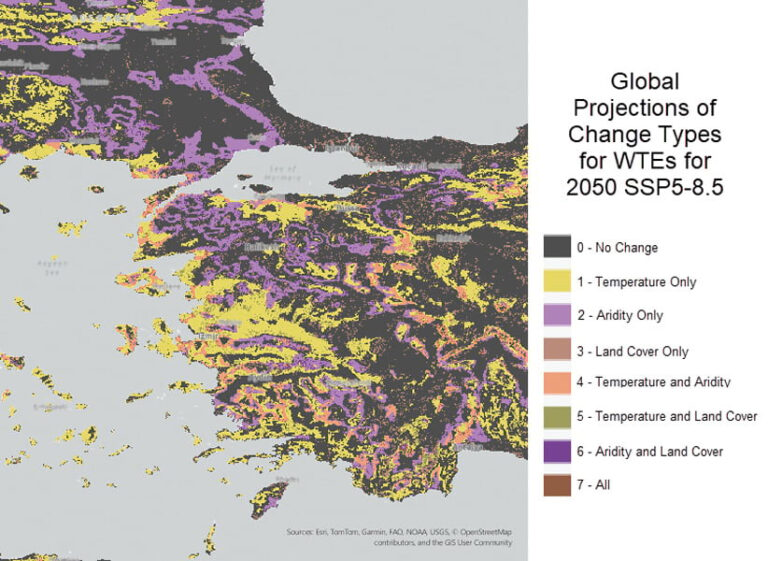
You can apply similar workflows to other types of crops for any region in the world using the 2050 Projected Terrestrial Ecosystems dataset from ArcGIS Living Atlas and the capabilities of ArcGIS Spatial Analyst in ArcGIS Pro.
Step 0: Set Up the Analysis Environment
Prior to doing any analysis, it is recommended that you ensure that all the steps of the analysis are done in the same analysis environment. Since we had data of different resolutions, extents, and coordinate systems, we set the cell size and snap raster to the coarsest resolution of 10 km, the analysis extent to the Olive Tree Dataset (10 km native resolution), and output coordinate system to the Global Projections of Change Types for WTEs for 2050 SSP5-8.5 (1 km native resolution). This ensured that we processed the correct extent and did not create any data that does not exist, thus introducing bias into our analysis.
Step 1: Reclassify Data
First, we reclassified the Global Projections of Change Types for WTEs for 2050 SSP5-8.5 data, decreasing the number of classes from seven to three, to differentiate between anthropogenic and climate change disturbances to create the Ecosystem Disturbance raster. Here, we assumed that any temperature or aridity change is classified as climate disturbance, and any land-cover change is anthropogenic disturbance. Classes that combine temperature or aridity with land cover are classified as anthropogenic, as the area has already changed and cannot be used for crop cultivation anymore. We used the Reclassify tool in the Spatial Analyst extension in ArcGIS Pro.
Step 2: View Overlap
Next, we combined the olive trees with the ecosystems disturbance raster to see the overlap of the disturbances with the olive tree distribution. Using the Combine tool, we assigned a unique combination to each overlap of ecosystem disturbance to olive tree distribution. There were 327 such unique combinations.
Step 3: Calculate Change
Now, using the Summary Statistics tool, we calculated the sum of the percentages of olive farms within each of these ecosystem disturbance zones as well as the zones that see no change over time.
Step 4: Create a Chart
To visualize our findings, we created a chart from the attribute table of the summary statistics. We clicked Create chart, chose Pie chart, and matched the colors of the chart with the map.
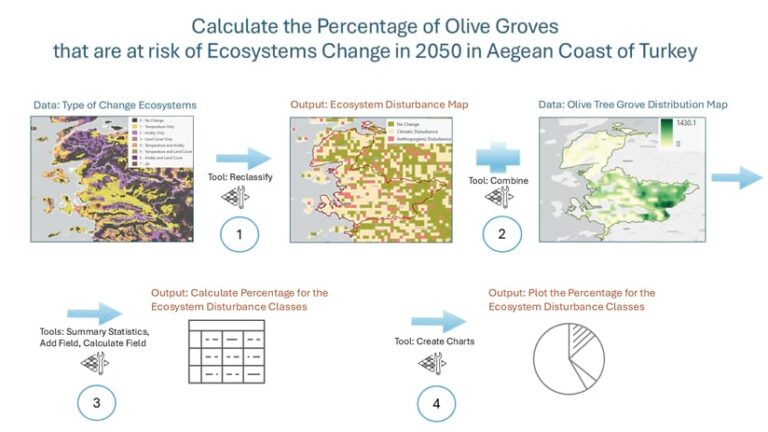
A Future for Olives
The projected terrestrial ecosystem changes are informed by models of future climate scenarios and the demands of expanding populations for settlement and agriculture. Models like ours do not prescribe what will happen but rather what may happen. The results are subject to change. However, according to our model, significant climate alterations that impact surrounding ecosystems underscore the necessity for adaptation.
The good news is that just because a region becomes less suitable for olive tree agriculture doesn’t mean it’s impossible to grow olive trees there. A loss of suitability means that more effort and resources are needed to grow viable and productive olive trees. Adapting to climate change in olive production could involve introducing or expanding irrigation systems or terracing hillsides to optimize growing conditions in milder temperatures. Farmers might use modular shades to protect olive trees from peak UV radiation, or apply treatments to ward off pests and diseases. Adjustments in orchard management could maximize water efficiency, improve soil nutrients, or utilize cover crops to enhance soil carbon retention.
In response to changing climates, olive cultivation may also shift to newly suitable areas, or farmers could explore different olive varieties that are more resilient under future conditions.
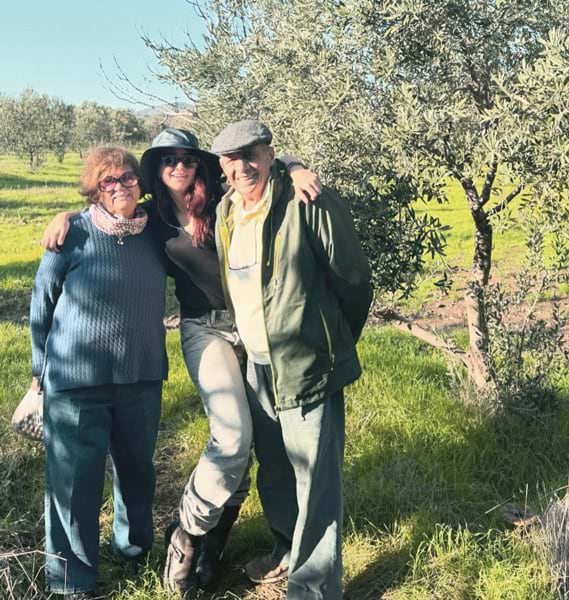
If we make the right decisions in managing the land with the future techniques of sustainable farming, regardless of potential climate and land-cover changes by 2050, we could save up to 88 percent of the olive trees on Turkey’s Aegean Coast. However, if we don’t, we risk losing 53 percent of these trees. (This is a projection based on our model, not a prediction. It describes what may happen, and the results are subject to change.) The Aegean Coast accounts for 80 percent of Turkey’s olive production, and since the country is one of the top three olive producers in the world, a failure to act will affect people far beyond just this region. Similar outcomes are likely in other olive-growing countries around the world.
As a result, we advocate for the integration of maps depicting potential ecosystem shifts into the planning of strategies meant to help us adapt to the effects of a changing climate, with a focus on the needs of local populations. In this analysis, we assessed areas where existing olive trees may be affected by these ecosystem changes, based on anticipated shifts in climate and the growing land requirements for human activities.
There is an urgent need for sustainable transformation in global food systems to support farmers, especially those who lack access to advanced agricultural technologies, in preparing for and adapting to changing climate and weather patterns. The world can no longer afford to delay these critical innovations.
Sidebar: World Terrestrial Ecosystems
Before you start using the Projected Change Types for the World Terrestrial Ecosystems (WTE) layer in your analysis, it is helpful to first understand the concept of WTEs. This will help you grasp what you are aiming to achieve and why.
In 2020, The Nature Conservancy (TNC), the United States Geological Survey (USGS), and Esri collaboratively developed a 250-meter-resolution WTE 2015 dataset. Terrestrial ecosystems are land-based environments where plants, animals, and microorganisms interact with each other and their physical surroundings. The WTEs are a set of 431 ecosystems conceptualized and mapped as distinct combinations of World Climate Region, generalized Hammond landform types, and European Space Agency (ESA) Global Land Cover for the year 2015.
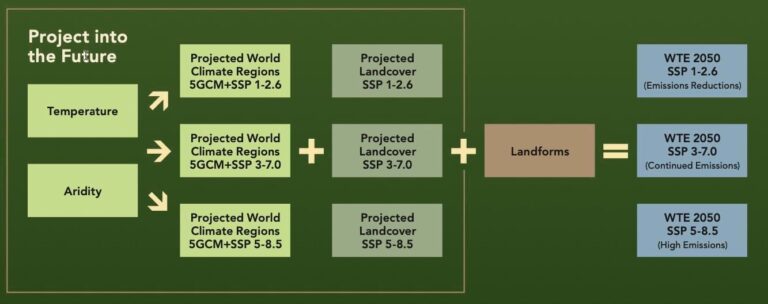
Temperature and aridity data from WorldClim 2 were used to produce 18 climate regions, following Intergovernmental Panel on Climate Change (IPCC) guidelines. Land cover was reclassified into eight classes, and landform data was derived from the 2010 digital elevation model (DEM), reclassifying the Hammond model landforms into four classes. This is the foundational layer that allows us to understand changes in terrestrial ecosystems when compared to the projected 2050 terrestrial ecosystems.
Last year, Esri collaborated with USGS, Conservation International, Wildlife Conservation Society, TNC, NatureServe, and Clark University. This collaboration extended the projections for WTE 2015 work by incorporating climate and shared socioeconomic pathway scenarios for 2050, and identifying the types, magnitude, and locations of changes in the distributions of Earth’s terrestrial ecosystems.
By leveraging global climate models, climate regions and land cover can be projected into the future. The latest global climate models, part of the Coupled Model Intercomparison Project Phase 6 (CMIP6), use a range of developmental and emissions scenarios known as Shared Socioeconomic Pathways (SSPs). SSPs represent narratives describing broad socioeconomic trends that could shape future society.
Three CMIP6 SSPs were selected to model three projected 2050 WTEs. The SSPs selected for the global ecosystem modeling were the emissions reductions pathway (SSP 1-2.6), the continued emissions pathway (SSP 3-7.0), and high emissions pathway (SSP 5-8.5).
The dataset used CHELSA 2.1 (1 km) climate models from CMIP6 instead of World Clim 2, following IPCC guidelines incorporated with three SSPs described above. For each SSP, modeled climate data was selected from five CHELSA-compiled CMIP6 GCMs: UKESM1-0-LL, MPI-ESM1-2-HR, MRI-ESM2-0, GFDL-ESM4, and IPSL-CM6A-LR. The combination of the five GCMs and three SSPs produced 15 bioclimate combinations with 19 classes of climate regions.
For the projected land-cover dataset, the existing models from Chen et al. (2022) were used. The Chen et al. models are 1 km spatial resolution global projections at five-year intervals from 2015 to 2100 for eight SSPs, including the three described above. The Chen et al. models harmonized global land-use data from the Land-Use Harmonization 2 (LUH2) model (Hurtt et al., 2020) and the ESA/CCI GLC data based on reclassifying the LUH2 outputs into 17 plant functional types (PFTs). They were then reclassified into eight classes.
The same landform dataset from 2015 WTE was used as the third parameter. In the case of the 2015 WTEs, the model combined three input layers to produce one ecosystem map. For the 2050 WTEs, however, the use of five GCMs each with three SSPs produced 15 climate region outputs, and the single model vegetation and land cover projections produced a total of three landcover outputs based on three SSPs. For the case of 2050 WTEs, the landform, landcover and the climate regions datasets included resolutions of 231, 927, 1157 meters respectively. These datasets were resampled to their lowest common denominator, and then aligned and combined. Finally, the dataset was resampled to approximately 1.25km cell size output to ensure a very small resampling error propagation.
The climate region, global vegetation and land cover, and landforms inputs were then combined to assign a WTE classification per SSP following the original WTE typology described in Sayre et al. (2020). The five 2050 WTEs for each SSP. To simplify outputs and derive one final, predominant WTE for each SSP, a majority rule was applied across all five 2050 WTEs per SSP to identify that which occurred most frequently. This produced three projected WTEs based on three SSPs and GCMs.
Using this data, the ecosystem type of every 1 km² land pixel on Earth was compared in 2015 to its projected type in 2050 under three scenarios, each representing varying trajectories of human behavior and the changing climate.